In DEMO, as in future fusion power plants, the deuterium-tritium nuclear fusion reactions will generate a large quantity of 14.1 MeV neutrons that will collide with the materials of the reactor vessel. The first wall, a combination of layers of different materials that aims to maximize the conversion of neutrons into thermal energy and breed tritium, will be critically exposed. Understanding the degradation of the mechanical properties throughout the reactor operational life is a key parameter to allow the design and eventual facility licensing by the corresponding nuclear authorities.
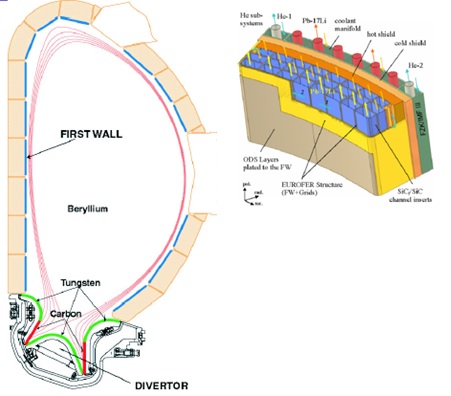
Inelastic collisions of neutrons with the nuclei in the structural materials over the threshold incident energy of around 3 MeV will transmute heavy nuclei, which can decay releasing p+ and α-particles. In turn, the elastic collisions are measured by NRT (conventional unit for structural damage under irradiation) displacements per atom (NRT dpa) with a cross section inversely proportional to the average displacement energy threshold for production of a Frenkel vacancy-interstitial atom defect pair in the material. Not all the materials will present the same NRT dpa under same neutrons bombardment, neither will all areas inside the reactor vessel undergo the same flux and spectrum of neutrons. In addition, NRT dpa do not take into account the time-evolution of radiation damage in the materials, such as recombination, migration, and coalescence of radiation defects, and in this sense they represent an atom-based approximate measure of the irradiation exposure of the material to the fusion neutrons. The generation of He and H from transmuted elements together with displacement effects in the materials lattice under fusion-like neutron bombardement has inherent uncertainties on the effects on mechanical and physical properties that can only be overcome through testing in as close as possible conditions.
IFMIF, the International Fusion Materials Irradiation Facility, will generate a neutron flux with a peak at around 14 MeV by (d,Li)n stripping reactions thanks to two parallel deuteron accelerators. Both deuteron beams will collide in a liquid Li screen with a footprint of 20 cm x 5 cm (see figure 1). The energy of the beam (40 MeV) and the current of the parallel accelerators (2 x 125 mA) have been tuned to maximize the neutrons flux (1018 m-2s-1) to get irradiation conditions comparable to those in the first wall of a fusion reactor in a volume of 0.5 x 10-3 m3 that will house around 1000 small specimens. This will allow the accurate determination of those properties considered critical (fatigue, fracture toughness, crack growth rate, creep and tensile stress) of suitable materials and allow the understanding of the degradation that will lead to design more radiation hard components.
The purposed-designed reduced activation ferritic-martensitic steels (RAFM) present typically around 80% of its constituents presenting an average displacement energy (Wigner energy) of 40 eV that can reach over 15 dpa/year in a full power operation fusion reactor. The accumulation of gas in the materials lattice is intimately related with neutron energy threshold at around 3 MeV; neutrons below these energies do not transmute the Fe nuclei and therefore gas is not efficiently generated. This is a major drawback for fusion materials testing in fission reactors as they present average n energies around 2 MeV. In turn, spallation sources present a neutron spectrum with long tails reaching the hundreds MeV range transmutation efficiency, especially for the lighter elements, which have a high potential in deteriorating mechanical properties. A comparison of IFMIF with other available neutron sources has been performed, where it is explained how the spectrum that IFMIF provides, reproduces accurately fusion reactors spectrum with a flattop near the 14 MeV peak and at intermediate energies, while the closest available spallation sources, ESS and XADS, are one order of magnitude lower in flux in these critical range of energies.
The IFMIF main expected contributions can be summarized in the following:
- provide data for the engineering design for DEMO
- provide information to define performance limits of materials and materials systems for DEMO and beyond
- contribute to the completion and validation of (existing) databases to gather and confirm data required for licensing and safety assessment
- contribute to the selection or optimization of different alternative fusion materials
- validate the fundamental understanding of radiation response of materials including benchmarking of irradiation effects modelling at length-scale and time-scale relevant for engineering application
- tests blankets and functional materials prior to or complementary to ITER test blanket modules